
Visitors Counter: Total Number to All Pages
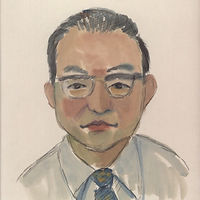
Favorite Papers
Tabata's Papers He likes
The link of each paper is to the source deposited at Tabata’s page of the Academia site, in the form of a re-edited post-print. You can find the published version by the DOI link at the top of the post-print.
The image above shows a portrait of Tabata drawn by K. Turu in 1977.
Backscattering of electrons from 3.2 to 14 MeV
T Tabata
Abstract: Monoenergetic electrons of energies from 3.2 to 14 MeV provided by a linear accelerator have impinged normally on thick targets. Backscattered electrons have been detected by an ionization chamber, the multiplication factor of which was calibrated with a Faraday chamber as a function of average energy per backscattered electron. Angular distributions of backscattered electrons measured for a total of seven targets, effectively semi-infinite and ranging in atomic numbers from 4 to 92, show a trend similar to Dressel’s result. However, the backscattering coefficients obtained are lower than his values and are consistent with those reported by other previous authors. Variations of angular distribution and backscattering coefficient with target thickness also have been investigated for Cu, Ag, and Au targets at an incident energy of 6.1 MeV. Some of the angular distributions observed were compared with results of a simple calculation proposed by the author, and an interpretation has been given that the relative contribution of sidescattering compared with that of diffusion increases with increasing energy in the region considered. Backscattering coefficients for the thinnest targets of lower atomic numbers level off toward a nonzero intercept similar to Cohen and Koral’s lower-energy result. This tendency is considered to be caused by the contribution of energetic secondary electrons. ...
Commentary: This paper was Tabata’s doctoral thesis. When our experiment was still at a preliminary stage, Harder and Ferbert reported the backscattering coefficients for energies from 8 to 22 MeV. It was lucky that our method of measurement was different from theirs. We were measuring the backscattering coefficients differential in scattering angle using the X-ray compensation type of ionization chamber developed by Van de Graaff et al., and considered it worth continuing our experiment. At the time our experiment was almost completed, Dressel reported the backscattering coefficients for energies from 0.5 to 10 MeV. His results were appreciably higher than the results obtained by the majority of previous authors, and he discussed that the discrepancy might be attributed to errors in the previous work. On the basis of our results, we were able to report timely that the cause of the discrepancy might be in Dressel’s work. This was confirmed by Harder and Metzger and by Ebert et al. Dressel himself carefully checked his experiment later and found that his errors had been caused by the peripheral halo of electrons that impinged on the target but was missed by the Faraday cup.
The reviewer of this paper reported: The author is a competent experimentalist, and the work appears to be carefully and painstakingly done.
​
​The figure below shows a comparison of our data with earlier ones.
​
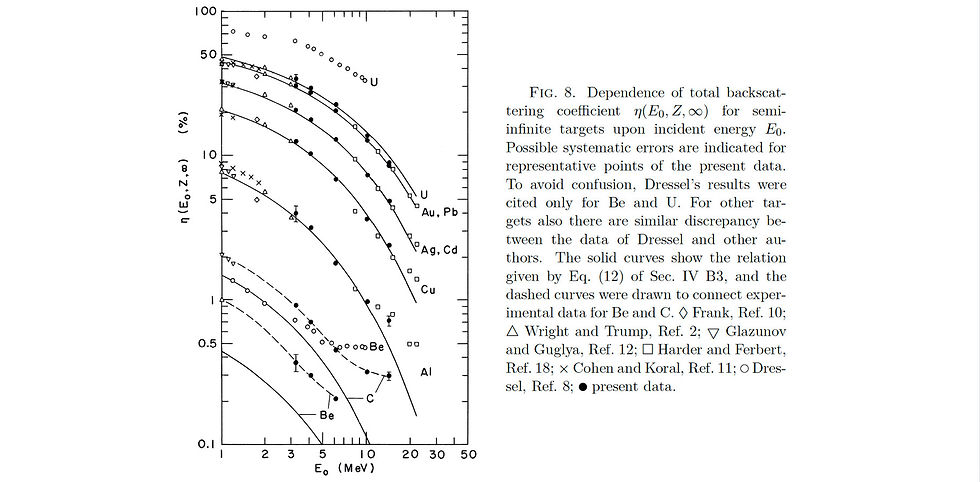
Charge distribution produced by 4- to 24-MeV electrons in elemental materials
T Tabata, R Ito, S Okabe, Y Fujita
Abstract: Charge-deposition distributions of monoenergetic electrons normally incident on thick absorbers of Be, Al, Cu, Ag, and Au have been measured with a thin collector moved through the absorber thickness. The measurements have been made at incident energies of 4.09, 7.79, 11.5, and 14. 9 MeV, and also at 23.5 MeV for absorbers other than Be. Values of the most probable charge deposition depth xm and the charge-deposition straggling w have been determined from the distributions observed. The ratio of xm to the theoretical mean range L of the incident electrons decreases with decreasing incident energy E0 and with increasing atomic number Z of the absorber, reflecting the effect of multiple-scattering detours of the primary electrons. The ratio w/L shows an increase with increasing E0 in the region of E0 approximately equal to or greater than 11 MeV for Al and in the entire energy region of the present experiment for Cu, Ag, and Au; this trend is considered mainly due to bremsstrahlung energy-loss straggling. [...] Some of the distributions are compared with the Monte Carlo results of Berger and Seltzer. Agreement between the experimental and calculated results is rather good except in the case of the Be absorber.
Commentary: Receiving a preprint of this paper, Berger wrote to Tabata: I like your paper very much; it is a substantial contribution, and very clearly written; your command of English is really excellent.
For the charge-deposition distribution of electrons of about 10-MeV energy in Be, there was a discrepancy between the experimental result of this work and the ETRAN Monte Carlo result by Berger and Seltzer. Comparing the EGS4 and ETRAN Monte Carlo results for the energy-deposition distribution of electrons in water, Rogers and Bielajew rediscovered similar discrepancies, i.e. higher penetrability of electrons in the ETRAN results. This led to the improvement of the energy-loss straggling algorithm used in the ETRAN code. Later comparison between the experimental and ITS-3.0 Monte Carlo charge-deposition distributions for Be showed good agreement (the ITS-3.0 code system used the same improved energy-loss straggling algorithm as ETRAN).
The figure below shows a comparison of the measured charge-deposition distribution with the Monte Carlo result of Berger and Seltzer, indicating a small discrepancy.
